- Magnetic Nanoparticles
A Web of Science search reveals thousands of articles published in the technologically and fundamentally important field of “interacting magnetic particles”, with a significant fraction of these dealing with how dipolar interparticle interactions in disordered particle systems may lead to spin-glass-like behaviour. In 2013 we presented a first model superspin-glass [1]: a nanoparticle ensemble exhibiting magnetic behaviour previously observed only in conventional spin-glasses*. This was achieved by preparing a material that “optimizes” (maximizes and yet limits the distribution of) dipolar interparticle interactions through tailoring of the spatial homogeneity and size distribution of nanoparticles. Namely, we realized a dense random assembly (close to the random-close-packed configuration, corresponding to the maximum possible particle volume fraction, as shown in the figure below) of higly uniform oxide nanoparticles. Recent progress in material science, which has facilitated the synthesis of large batches of identical (within less than 2-3 % of the mean diameter) nanoparticles, was instrumental in this realization of what may be considered the closest nanoparticle analogue (driven by dipolar interactions) of a conventional magnetically ordered state (driven by exchange) reported to date.
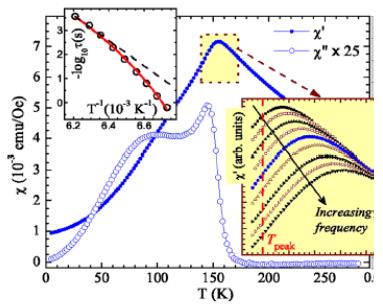
More over, by comparing our model compact system with other, less concentrated, ensembles of the same particles coated with silica shells [2] (see TEM micrograph below), as well as with other compacts made with uniform particles of different sizes [3], we have provided answers to the fundamental question as to the relative importance of interparticle superexchange versus dipolar interaction between oxide magnetic particles in direct physical contact. In a broad sense, the study of interparticle interactions, sometimes leading to superspin-glass or to superferromagnetic behavior, is partly motivated by the technological applications of dense magnetic NP ensembles, ranging from magnetic storage to cancer therapies based on magnetic particles and sometimes relying on interparticle interactions. The experimental techniques employed in this research line are, among others, SQUID magnetometry, TEM and HRSEM microscopy, and small-angle x-ray scattering (SAXS).
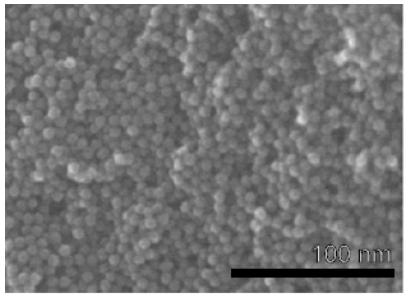
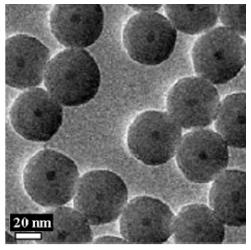
- Exchange bias in nanostructures
Exchange coupling between an antiferromagnet (or a random magnet) and a nanoscale ferro- or ferrimagnet may yield a significantly enhanced magnetic rigidity (anisotropy) of the latter. This is most commonly observed as a horizontal shift in the hysteresis loop measured under certain protocols (the shift is referred to as “exchange bias”). Exchange bias is extensively exploited in the so-called spin-valves, the magnetoresistive reading sensors in everyday hard disk write/read heads; however, and despite being known since decades, it is still a challenging effect to understand from a fundamental point of view. In the ApNano group we explore exchange bias in a variety of nanostructures, including multilayered thin films [1] and nanoparticles [2]. In the latter case, this research line thus deals with “intraparticle (core-shell) interactions”, which complements the line on “interparticle interactions”. In most real systems of the ubiquitous Fe-oxide nanoparticles, both intra- and inter-particle interactions are present.
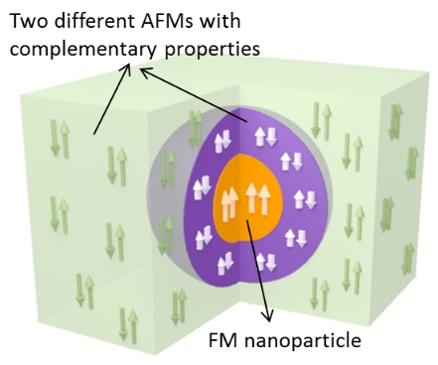
In the context of magnetic nanoparticles, exchange coupling has been suggested as a strategy to delay the superparamagnetic limit. If one takes a fridge magnet (or, for the same purpose, a bit of information from our hard drives) and shrinks it sufficiently (down to the nanometer range), the thermal energy in the room will be enough to make the north and south poles flip millions of times per second (i.e., the magnet will be in the superparamagnetic regime). This renders the nanomagnets useless, since they can no longer retain a fixed magnetization in the absence of applied magnetic field, which is the basis of permanent magnets and magnetic recording. In other words, the miniaturization race in certain magnetic devices is doomed to end at the “superparamagnetic limit” abyss. A successful approach to push this limit further down in size was reported years ago in the journal Nature [3], in which small ferromagnetic nanoparticles were stabilized by coupling them to an antiferromagnet. The downside was that the stabilizing effect ceased at a still low 15 ºC, which makes the effect impractical. In 2015, the ApNano group (in collaboration with researchers at Barcelona and Grenoble) reported the preparation of a superior artificial antiferromagnet (capable of stabilizing small nanomagnets beyond 120 ºC) through the convenient hybridization of the properties of two naturally occurring antiferromagnets (cobalt oxide and nickel oxide) via a proximity mechanism, and proposed a model that explains the nature of such an effect [2].
[1] Influence of spacer layer morphology on exchange bias properties of reactively sputtered Co/Ag multilayers
P. S. Normile et al., Physical Review B 76, 104430 (2007).
[2] High temperature magnetic stabilization of cobalt nanoparticles by an antiferromagnetic proximity effect
J.A. de Toro et al., Physical Review Letters 115, 057201 (2015).
[3] Beating the superparamagnetic limit with exchange bias
V. Skumryev et al., Nature 423, 850 (2003).
- Education & Communication
Diversos miembros del grupo hemos llevado experiencias de Física a las Semanas de la Ciencia, organizadas por la UCLM, y participado en las Noches de los investigadores, en Pint of Science y en las Jornadas de Puertas Abiertas del Instituto Regional de Investigación Científica.
José A. De Toro ha sido también colaborador del portal web de divulgación www.cienciaes.com y participado en cursos de verano en distintas universidades. Pablo Muñiz y otros profesores del grupo han visitado institutos de secundaria bajo la organización del Departamento de Física Aplicada para dar charlas divulgativas.
Además, nuestro interés por la enseñanza de la Física general nos ha llevado a realizar estudios que han dado lugar a una publicación en una revista indexada del área Physics Education.
– Jose A. De Toro, Gabriel F. Calvo, and Pablo Muñiz, “Two-Dimensional Crystallography Introduced by the Sprinkler Watering Problem.” European Journal of Physics 33(1), 167–177 (2012).
- Estrategia de Especialización Inteligente de Castilla-La Mancha (RIS3 CLM)
En cuanto a la organización y participación en actividades de innovación docente, varios miembros del grupo hemos participado en cursos de Enseñanza de la Física al profesorado de Educación Secundaria y Bachillerato que han sido organizados por la Junta de Comunidades de Castilla-La Mancha y/o la UCLM.
Además, Pablo Muñiz ha sido organizador de la XXXII Reunión Bienal de la Real Sociedad Española de Física y 19º Encuentro Ibérico de Enseñanza de la Física (Ciudad Real, 7-11 de septiembre de 2009). Varios miembros del grupo presentaron contribuciones en este evento.
- Estrategia de Especialización Inteligente de Castilla-La Mancha (RIS3 CLM)
Las líneas de investigación del grupo Apnano de la UCLM están en consonancia con los objetivos del Programa Operativo Plurirregional de España y de la Estrategia de investigación e innovación para la especialización inteligente RIS3 de Castilla-La Mancha. Nuestra actividad investigadora financiada está orientada a las aplicaciones de energía y de sensores con nuevos materiales magnéticos de mucha incidencia en diversos sectores estratégicos, en particular los sectores estratégicos 4 (Aeronáutica) y 5 (Medio Ambiente y Energía) de la RIS3 de Castilla La Mancha. Además de la generación de conocimiento, nuestra actividad ha estado también dirigida a la transferencia de resultados, con patente y una licencia de patente productiva. En la región y en el territorio nacional hay empresas con las que colaborar en caso de volver a patentar, quizás un nuevo método de síntesis, nueva instrumentación o la propuesta de un nuevo sensor magnetorresistivo o de magnetoimpendancia. Nuestro grupo tiene actualmente dos ejes de actividad investigadora, que a continuación encuadramos en dichos sectores estratégicos.
– Los nuevos sensores en relación a los sectores estratégicos 4 y 5:
Los sensores magnetorresistivos y de magnetoimpedancia están presentes en muchos sectores, algunos muy avanzados como la aviónica, la robótica y los sistemas cognitivos, otros de mucha implantación como la generación y/o conversión de energía, vehículos, telefonía móvil, seguridad y otras industrias. Los materiales sensores que estudiamos son punteros, pues su diseño aúna una robusta geometría de nanohilos planos cuasi-paralelos, un intenso efecto magnetorresistivo, y marcadas anisotropías controlables, permitiendo así combinar una altísima sensibilidad tanto a la intensidad como a la dirección del campo magnético. Además, no menos importante es la obtención de estos sistemas materiales sobre un soporte flexible, ligero y transparente, pues así son entonces de interés para dispositivos electrónicos flexibles y otras tecnologías emergentes.
– Los nuevos imanes permanentes en relación a el sector estratégico 5:
De otro lado, la necesidad de nuevos imanes permanentes con mejores propiedades es enorme, al usarse sistemáticamente en los alternadores de las turbinas de aerogeneradores y centrales convencionales, así como en los motores de coches eléctricos. Una mejora del 1% en la conversión energética resultaría, sólo en la Unión Europea, en ahorros anuales de aprox. 1000 millones de euro y en una reducción de las emisiones de CO2 equivalente a millones de toneladas de carbono. Pero no sólo interesa un nuevo material magnético con mejor eficiencia energética sino también que no contenga materiales críticos. En particular, la reducción o eliminación de tierras raras en las turbinas eólicas tendría especialmente un impacto en Castilla-La Mancha, región donde esta industria está señalada entre las prioridades S3P de la comisión europea. Debemos apuntar que cada turbina eólica requiere el empleo de unos 600 kg de Nd2Fe14B por megavatio de potencia instalada (por tanto, cientos de kilogramos de neodimio; un material crítico para la UE por su importancia tecnológica y riesgo de suministro).